Feature
More than a century ago, scientists exploring phenomena at exceptionally minute scales began reporting strange results that seemed to contradict the established laws of physics: Particles that behaved like waves. Waves that behaved like particles. Discrete values of quantities that surely should instead be continuous, like energy. The implications of these new discoveries were so bizarre, so far-removed from everyday experience, that even one of the "fathers of quantum theory," Max Planck, initially wondered whether they were purely mathematical tricks with no basis in physical reality.
Today, we accept these ideas as fundamental principles of quantum science—the study of nature at its smallest scale. As our knowledge of the quantum world has advanced in recent years, Caltech engineers and applied scientists are increasingly asking what we can do with this new information. In other words, explains Oskar Painter (MS '95, PhD '01), John G Braun Professor of Applied Physics, "what started out as a physics mystery has now moved into the field of engineering." To further explore this frontier of engineering, ENGenious sat down with Painter and three of his Engineering and Applied Science colleagues. The roundtable discussion with Painter, Stevan Nadj-Perge, Andrei Faraon, and Austin Minnich, inspired this article.
Quantum engineering—as this nascent field is now known—is a rapidly evolving area "in which quantum properties are essential to how a device or technology operates," says Stevan Nadj-Perge, Assistant Professor of Applied Physics and Materials Science. It's a relatively new term, but the field itself has deep roots in disciplines such as computer science, electrical engineering, materials science, and applied physics. Quantum engineering is now finally coming into its own. "In the same way that electrical engineering split from physics more than a hundred years ago, I think soon quantum engineering will split from more-classical engineering and physics," says Andrei Faraon (BS '04), Professor of Applied Physics and Electrical Engineering.
From quantum computers, currently quite specialized, but posited to eventually outshine today's general-purpose super-computers, to novel devices for probing tough cosmological mysteries, the potential applications of quantum engineering are groundbreaking and far-reaching. But, as Austin Minnich, Professor of Mechanical Engineering and Applied Physics, notes, "it's not just about industrial and techno- logical applications. It's really about doing science in a completely different way."
A Quantum Leap Forward
In many ways, quantum engineering has already had a tremendous impact on the world, even before the term quantum engineering was coined. Technologies like lasers, transistors, electron microscopy, and magnetic-resonance imaging were all developed based on concepts of quantum science. However, previous work does not depend on complex quantum interactions between particles that can result in even more bizarre emergent properties. Researchers have long known about these behaviors, but only recently have the science and technology advanced to a point where it's conceivable to harness such behaviors.
"The grand challenge of quantum engineering in the 21st century is how to make use of the more subtle aspects of quantum theory—concepts like entanglement—which people like Bohr, Einstein, Heisenberg, and Schrödinger really struggled with," says Painter. Successful utilization of quantum properties such as entanglement (the way particles influence one another's states) and superposition (the ability of individual particles to exist in two states at once) is what is enabling a giant leap forward in quantum engineering today.
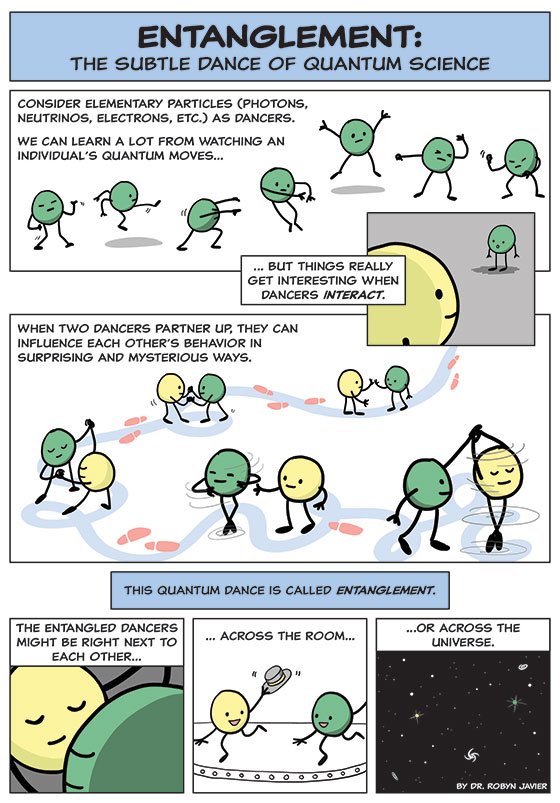
Innovative and ground-breaking work is happening at Caltech, thanks to the Institute's unique strengths across the engineering and applied science disciplines and the EAS faculty's deep connections to the sciences. Caltech has a rich history of breakthroughs in quantum science (consider the Nobel-winning work of Richard Feynman on quantum electrodynamics and Linus Pauling on quantum chemistry), and now it has become a leading incubator for quantum engineering as well.
Nadj-Perge is one of several faculty members working at the leading edge of quantum engineering. His research group is particularly interested in new materials for quantum computers. "One of the bottlenecks of doing quantum computation is that the exotic materials needed to push the field further have basically not yet been invented," he explains. "All the practical materials that we have around have some limitations—and we don't even fully understand those limitations." Determining what the ideal materials would look like, and how to develop them, is therefore a major focus of his research. The results could greatly improve quantum devices and underlie the design of future quantum computers.
Another challenge in developing quantum systems is the need to take extremely quiet quantum signals and amplify them to the everyday scale. That is, we need to produce an output that "we can lay our grubby, classical hands on," to borrow a phrase from renowned physicist and Caltech alumnus Carlton Caves (PhD '79). Minnich's group is addressing this challenge by improving the semiconductor-based micro- wave amplifiers often used in quantum systems. "We think we have a way to reduce the noise of those amplifiers to the lowest level physically possible," says Minnich. "If we're successful, it would greatly enhance our ability to probe nature at its very fundamental quantum limits."
Minnich is also exploring quantum engineering from a theoretical perspective, using classical computers to simulate quantum phenomena. Whereas experimental approaches can produce data that might be interpreted in multiple ways, simulation can provide a more precise way of refining the predictions of a theory. Consider a difficult problem like the Schrödinger equation, which can't be solved exactly. "Numerical tools go beyond what we can do with pen and paper by taking this equation and solving it under certain approximations," explains Minnich. "This essentially provides a simulation of an experiment." Such an approach can be useful, for example, when interpreting the emergent "quasi-particles" that result from the interactions of elementary particles in a material.
Light is another commonly used tool in quantum engineering, and it is the primary workhorse in Faraon's laboratory. Operating near the fundamental limit of light- matter interactions, his group uses photons traveling through optical fibers to probe single atoms. The insights gleaned from his research could have major implications for quantum computing. Faraon notes an additional benefit of working with light: "It allows you to control quantum systems that are actually spaced quite far apart. Once a photon is in an optical fiber, the distance it travels—whether a meter or many kilometers—doesn't really matter." That means it may be possible to create inter- connected quantum systems that are delocalized over a very large area.
Painter's research interests cover a broad swath of quantum engineering, from optical materials and devices to superconducting quantum circuits. One particular area of focus, however, is exploring what so-called "early-stage quantum computers" can do. "Quantum systems today still make too many errors to be fully useful," says Painter, "but we can already start to build complex circuits capable of doing fairly mature computations." Understand- ing how to best use quantum computers as they currently exist will undoubtedly facilitate important applications of this powerful technology in the near term. Looking further ahead, such insights will lay a critical foundation for the future as quantum computers continue to evolve.
Bringing It All Together
Quantum engineering is a field driven by convergence—the convergence of disciplines that initially led to its creation, and now the convergence of engineering and technological advances that is ushering in a new era of devices. Thanks to the intensely collaborative and interdisciplinary environment at Caltech, faculty like Nadj-Perge, Minnich, Faraon, and Painter can explore synergistic connections among their areas of expertise.
For example, Minnich's efforts to improve microwave amplifiers are directly relevant to researchers like Painter whose experiments rely on microwave-based quantum systems. Microwave systems can, in turn, be connected with other types of quantum systems. In particular, Painter and Faraon are interested in connecting their microwave systems and light systems to enable quantum communication across modalities. The instantaneous transmission of quantum information from one location to another is known as quantum teleportation. This phenomenon was first observed 20 years ago at Caltech using a purely optical system, but achieving quantum teleportation between two completely different types of quantum systems is an intriguing possibility.
A convergence of solutions will also likely be required to overcome the problem of "parasitic entanglement," a term referring to the way experimental systems can become entangled with other objects in the environment, thus leaching away the subtle information researchers are trying to observe. Painter points to research developing new types of computational algorithms that can accommodate more imperfections, allowing for the reality of parasitic connections while salvaging enough quantum information to be useful. Meanwhile, Nadj-Perge seeks to overcome the problem altogether by exploring materials with so-called topological protection that intrinsically "hides" information from the environment.
Critical contributions to this emerging field are not limited to academia. Progress in quantum engineering to date has been greatly facilitated by work in industry. Google, for example, has become heavily involved in quantum computer research and development, recently announcing a 53-qubit system that performed a sampling calculation in under 4 minutes that would have taken the fastest super-computers an estimated 10,000 years to perform. Revised estimates are still being made that show this time can be substantially reduced with specialized memory-usage algorithms, but the writing is on the wall regarding the challenges faced by classical computers in keeping up with new quantum computing hardware. Painter notes that each of the academic and industrial worlds brings its unique strengths—flexibility and freedom to do basic exploration on the academic side, and the capacity for sustained investment and large-scale efforts on the industry side. "Quantum engineering is such a hard thing that we're going to need new breakthroughs at every level," he says. "And so that tandem force of industrial investment along with academic investment is key."
Quantum Engineering in Action
Perhaps the most tangible evidence of recent progress in quantum engineering is the increased size and complexity of quantum devices. Just a couple years ago, the most advanced quantum systems contained about 5 qubits (a qubit, short for "quantum bit," is the fundamental building block of quantum systems). Today, researchers have successfully engineered systems with 50 qubits or more. "That might not sound like much—going from 5 to 50—but every time we add a single qubit, we double the complexity of the system," explains Painter. "So, it's really exponential improvement, and it's an enormous breakthrough."
With these advances, researchers have established a new era that is punctuated by various feats of "quantum supremacy." Quantum supremacy, a term coined by John Preskill, Richard P. Feynman Professor of Theoretical Physics, refers to the first use of a quantum computer to solve a problem that is effectively impossible to solve using classi- cal computers. Achievement of quantum supremacy by various devices will result in a string of unique milestones in quantum engineering, parallel to other ‘firsts' such as the first flight or the first earth-orbiting satellite. These events heralded tipping points that led to vast techno- logical and societal changes.
Tackling impossibly difficult problems via quantum computing may open the floodgates of insight across many scientific fields. "Being able to solve these very difficult quantum simulation problems can actually help us understand our universe better," says Minnich. Take black holes, for example. The question of what happens to information that falls through a black hole's event horizon has vexed physicists for years. According to Minnich, answers may come from measuring how reliably information can be retrieved via "quantum teleportation" across a network of qubits—which provides a window into the type of information scrambling that may occur within a black hole. Cracking this cosmic mystery is just one potential example of the power of quantum computation, and quantum computation is just one area of quantum engineering.
Another major area of quantum engineering is quantum metrology—the development of sensors and measurement devices that use quantum properties to deliver unprecedented precision and sensitivity. Consider the Laser Interferometer Gravitational- Wave Observatory (LIGO), which used extremely sensitive equipment to achieve the first-ever detection of gravitational waves (signals so faint that Einstein thought they would never be possible to observe). Yet even this incomparably precise undertaking was ultimately limited by quantum mechanical properties of the massive mirrors used to measure displacement. Quantum engineering, on the other hand, can push beyond the so-called standard quantum limits of measurement, surpass- ing LIGO's current capabilities and enabling fundamentally new types of astronomy.
Quantum measurement devices may provide a path forward in high-energy physics, where many current experiments require particle accelerators with a footprint the size of a small city. "We can't just keep making larger and larger energy colliders," observes Painter, "but rather we can make increasingly precise measurements in a laboratory setting." Imagine experiments currently conducted in massive particle accelerators like the Large Hadron Collider (LHC) but carried out in a standard laboratory setting.
Other major areas of quantum engineering include development of quantum materials and communication. The possibilities touch literally every area of science and technology. Nadj-Perge sums it up this way: "Quantum engineering is definitely where the next revolution in science and engineering will happen."
Training for the New Frontier
In industry, demand for quantum engineers is already surging. "Companies like IBM and Google are telling us they need quantum engineers now, but the workforce largely doesn't exist yet," says Minnich. Fortunately, Caltech has long been at the forefront of education in quantum science and is now leading the way in development of rigorous training for quantum engineers.
For example, Caltech is launching a minor in quantum science and engineering for graduate students. The program expands upon previously developed curriculum, and will offer courses on quantum hardware, devices, and techniques, as well as near-term quantum computing. Its formal structure ensures students receive the comprehensive education needed for a career in quantum engineering, and the degree itself provides clear recognition of the students' specialized training—a key advantage when applying for jobs. Faraon sees the minor as an important first step, adding that "as the field continues to grow, we will likely offer master's and PhD degrees in the future."
Naturally, research opportunities in quantum engineering at Caltech are not limited to graduate students. Undergraduate students have access to specialized courses and the Summer Undergraduate Research Fellowship in Experimental Quantum Science and Technology (QuantumSURF). About a dozen faculty members participate in QuantumSURF, including Minnich, Painter, Nadj-Perge, and Faraon.
In the coming years, the true impact of all these efforts will unfold as Caltech's students and postdoctoral scholars move on to careers in quantum engineering. Whether that means blazing new intellectual trails in academia, tackling the toughest problems at leading tech companies, or creating start-ups that define entirely new quantum engineering-based industries, Caltech students are uniquely equipped for success. "Despite Caltech's small size, its students tend to have an outsized impact because they're the people who go on to create new ideas and become leaders in the field," says Painter. In this new age of quantum engineering, he predicts that "Caltech will again be very influential as our students grow."
Andrei Faraon is Professor of Applied Physics and Electrical Engineering. Austin Minnich is Professor of Mechanical Engineering and Applied Physics. Stevan Nadj-Perge is Assistant Professor of Applied Physics and Materials Science. Oskar Painter is the John G Braun Professor of Applied Physics. Robyn Javier is a STEM Communication Specialist and Lecturer in the Caltech Division of Engineering and Applied Science.